Strange mathematical term changes our entire view of black holes
Black holes keep getting weirder.
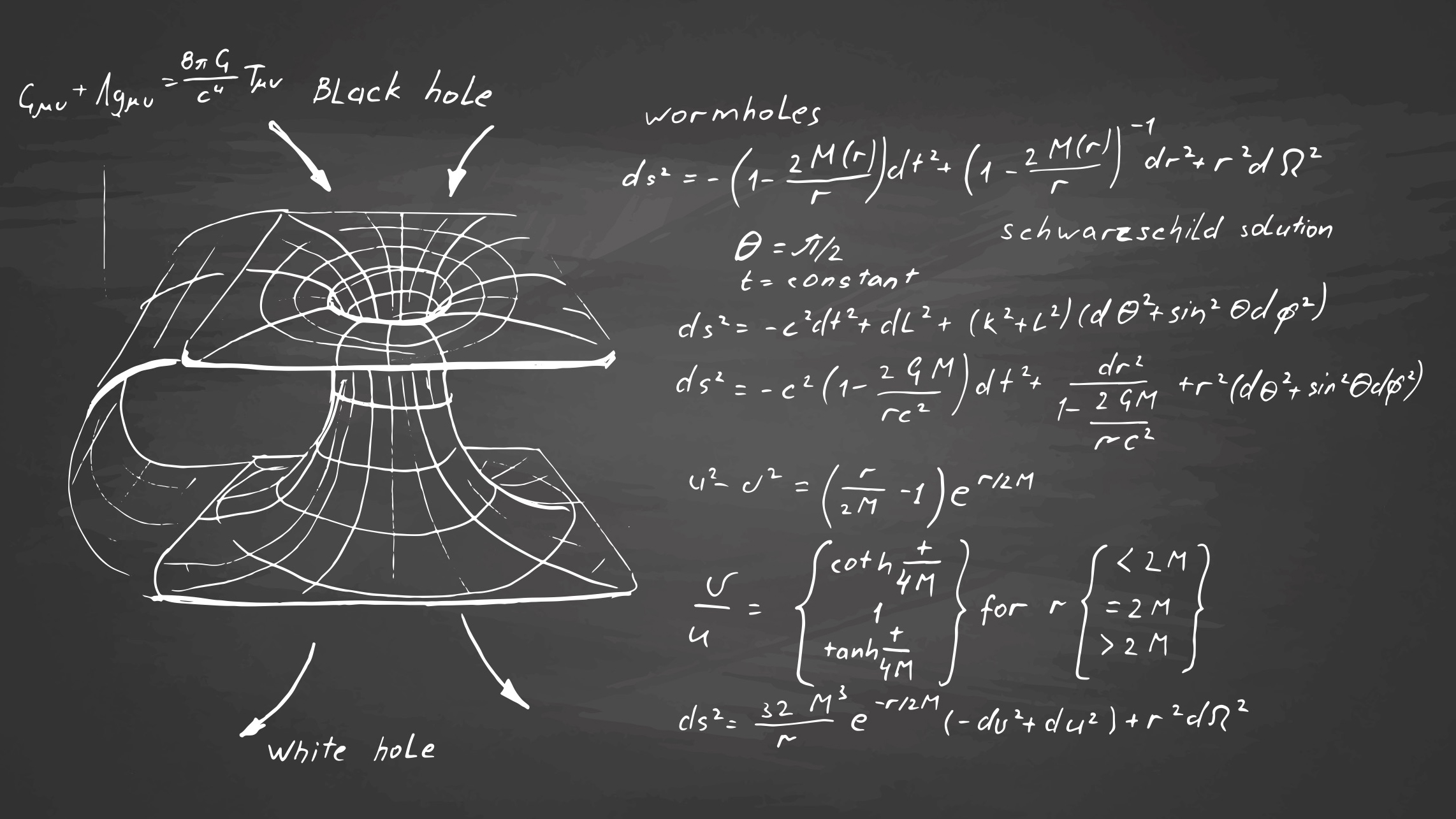
Black holes are getting weirder by the day. When scientists first confirmed the behemoths existed back in the 1970s, we thought they were pretty simple, inert corpses. Then, famed physicist Stephen Hawking discovered that black holes aren't exactly black and they actually emit heat. And now, a pair of physicists has realized that the sort-of-dark objects also exert a pressure on their surroundings.
The finding that such simple, non-rotating "black holes have a pressure as well as a temperature is even more exciting given that it was a total surprise," co-author Xavier Calmet, a professor of physics at the University of Sussex in England, said in a statement.
Related: 8 ways we know that black holes really do exist
Calmet and his graduate student Folkert Kuipers were examining quantum effects near the event horizons of black holes, something that is fiendishly hard to pin down. To tackle this, the researchers employed a technique to simplify their calculations. As they were working, a strange term appeared in the mathematics of their solution. After months of confusion, they realized what this newly discovered term meant: It was an expression of the pressure produced by a black hole. Nobody had known this was possible before, and it changes the way scientists think about black holes and their relationships with the rest of the universe.
Hawking's engine
In the 1970s, Hawking became one of the first physicists to apply quantum mechanics to try to understand what happens at the event horizon — the area around a black hole beyond which nothing, not even light, can escape. Prior to this work, everyone had just assumed that black holes were simple objects. According to general relativity, the theory of gravity that first suggested black holes could exist, there is nothing at all remarkable about the event horizon. The event horizon is the "boundary" of a black hole, defining the region where exiting the black would require traveling faster than light. But it was just an imaginary line in space — if you happen to cross it, you wouldn't even know you did, until you tried to turn around and leave.
Related: 8 ways you can see Einstein's theory of relativity in real life
Hawking changed all that. He realized that quantum foam, which refers to a sea of particles constantly popping into and out of existence in the vacuum of space-time, can affect that simplistic view of the event horizon. Sometimes pairs of particles appear spontaneously from the empty vacuum of space-time, then annihilate each other in a flash of energy, returning the vacuum to its original state. But when this happens too close to a black hole, one of the pair can get trapped behind the event horizon and the other escapes. The black hole is left holding the energy bill for the escaped particle, and so it has to lose mass.
Sign up for the Live Science daily newsletter now
Get the world’s most fascinating discoveries delivered straight to your inbox.
This process is now known as Hawking radiation, and it's through these calculations that we discovered that black holes aren't entirely, 100% black. They glow a little. This glow, known as "blackbody radiation," means they also have heat and entropy (also called "disorder") and all the other terms we usually apply to much more mundane objects like refrigerators and car engines.
An effective technique
Hawking focused on how quantum mechanics affected the vicinity of a black hole. But that's not the entire story. Quantum mechanics doesn't include the force of gravity, and a complete description of what's going on near event horizons will have to include quantum gravity, or a description of how strong gravity acts at teeny tiny scales.
Since the 1970s, various physicists have tried their luck both at developing a theory of quantum gravity and at applying those theories to the physics of the event horizon. The latest attempt comes from this new study by Calmet and Kuipers, published in September in the journal Physical Review D.
"Although the pressure exerted by the black hole that we were studying is tiny, the fact that it is present opens up multiple new possibilities, spanning the study of astrophysics, particle physics and quantum physics."
Xavier Calmet
"Hawking's landmark intuition that black holes are not black but have a radiation spectrum that is very similar to that of a black body makes black holes an ideal laboratory to investigate the interplay between quantum mechanics, gravity and thermodynamics," Calmet said.
Without a full theory of quantum gravity, the duo used an approximation technique called effective field theory, or EFT. This theory assumes gravity at the quantum level is weak — an assumption that allows you to make some progress in the calculations without everything falling apart, as happens when gravity in the quantum regime is modeled as extremely strong. While these calculations will not reveal the full picture of the event horizon, they may deliver insights around and inside the black hole.
"If you consider black holes within only general relativity, one can show that they have a singularity in their centres where the laws of physics as we know them must break down," explained Calmet. "It is hoped that when quantum field theory is incorporated into general relativity, we might be able to find a new description of black holes."
Here comes the pressure
Calmet and Kuipers were exploring the thermodynamics of black holes using EFT in the vicinity of the event horizon when they noticed a strange mathematical term pop up in their equations. At first, the term completely stumped them — they didn't know what it meant or how to interpret it. But that changed during a conversation on Christmas day, 2020.
They realized that the term in the equations represented a pressure. An actual, real pressure. The same pressure that the hot air exerts inside of a rising balloon, or pressure on a piston inside the engine of your car.
"The pin-drop moment when we realised that the mystery result in our equations was telling us that the black hole we were studying had a pressure — after months of grappling with it – was exhilarating," recalled Kuipers.
That pressure is almost absurdly tiny, less than 10^54 times smaller than standard air pressure on the Earth. But it's there. They also found that the pressure can be positive or negative, depending on the particular mix of quantum particles near the black hole. A positive pressure is the kind that keeps a balloon inflated, while a negative pressure is the tension you feel in a stretched rubber band.
Their result extends the idea of black holes as thermodynamic entities that have not just temperature and entropy, but also pressure. Because their work only models weak quantum gravity and neglects strong gravity, it can't completely explain the behavior of black holes, but it's an important step.
"Our work is a step in this direction, and although the pressure exerted by the black hole that we were studying is tiny, the fact that it is present opens up multiple new possibilities, spanning the study of astrophysics, particle physics and quantum physics," Calmet concluded.
Originally published on Live Science.
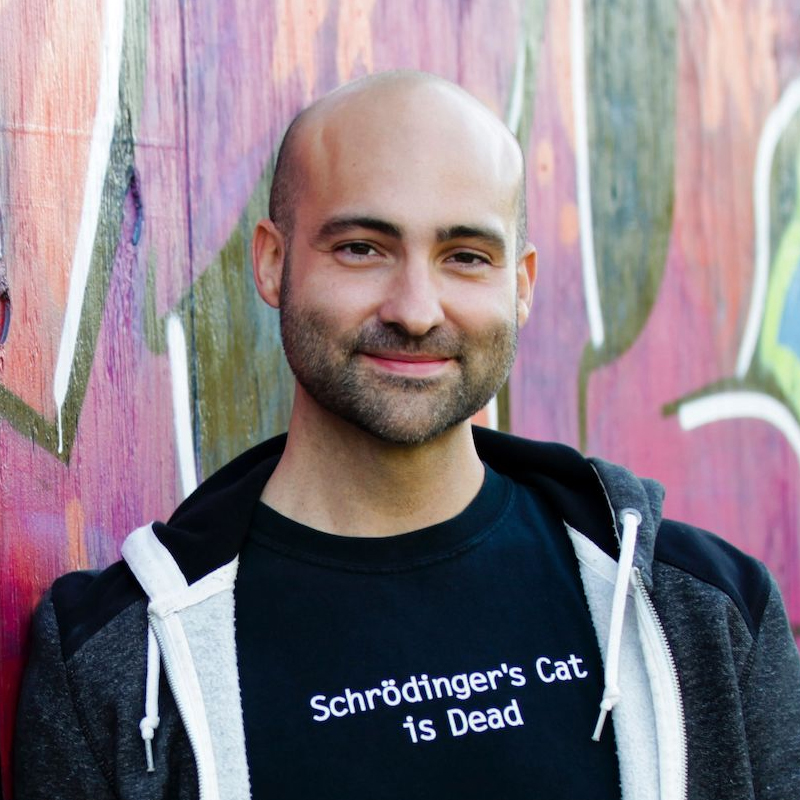
Paul M. Sutter is a research professor in astrophysics at SUNY Stony Brook University and the Flatiron Institute in New York City. He regularly appears on TV and podcasts, including "Ask a Spaceman." He is the author of two books, "Your Place in the Universe" and "How to Die in Space," and is a regular contributor to Space.com, Live Science, and more. Paul received his PhD in Physics from the University of Illinois at Urbana-Champaign in 2011, and spent three years at the Paris Institute of Astrophysics, followed by a research fellowship in Trieste, Italy.