450-mile-wide solid metal ball forms Earth's innermost core, earthquake waves reveal
Scientists calculated the diameter of Earth's innermost core using earthquake waves that bounced through the planet 'like ping-pong balls.'
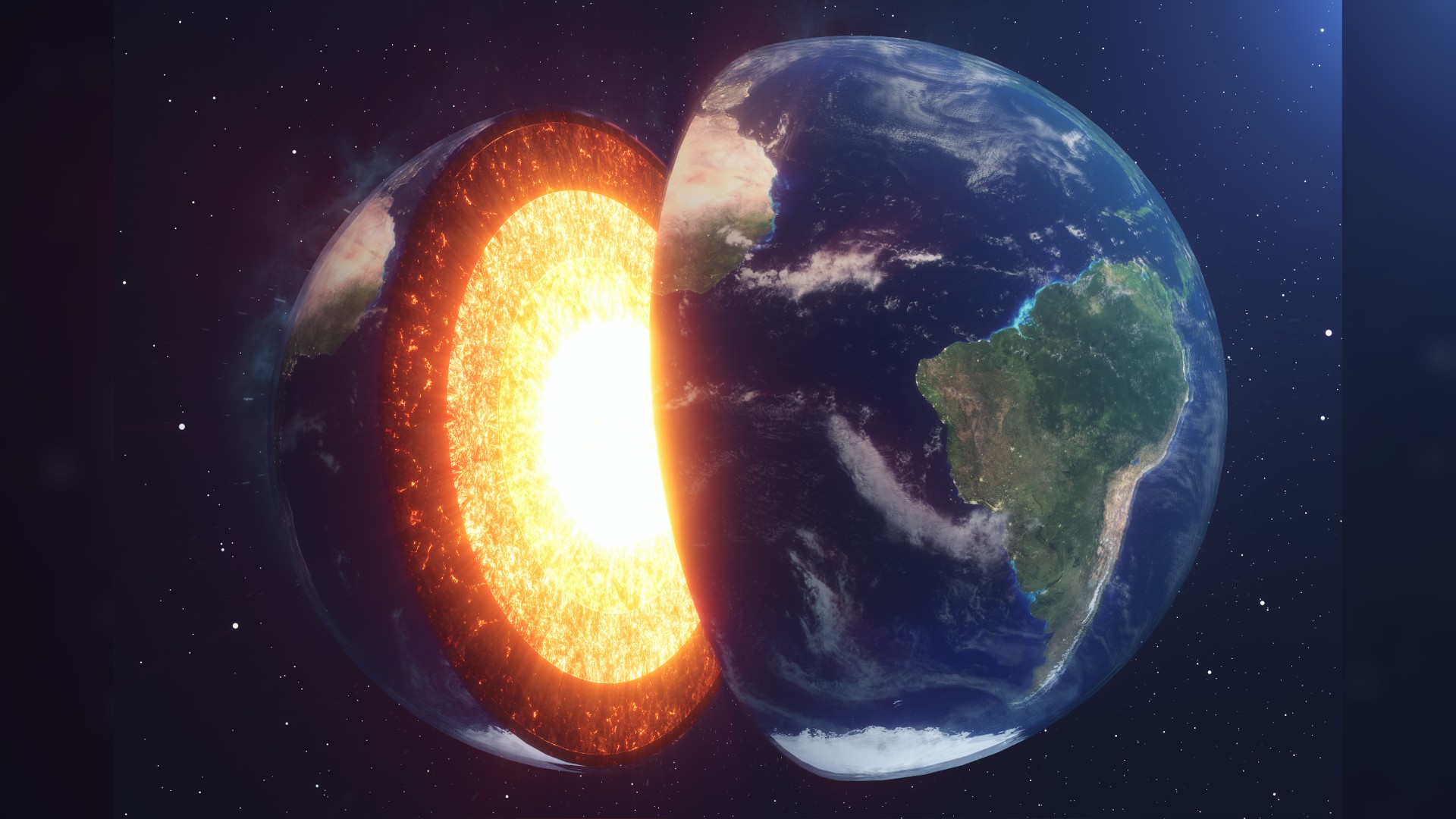
Scientists have harnessed powerful waves from earthquakes to measure Earth's innermost layer and found that our planet's center is a 450-mile-wide (725 kilometers) ball of solid iron-nickel alloy.
Previously, many researchers believed that Earth had four distinct layers — the crust, the mantle, a liquid outer core and a solid inner core. But in the past couple of decades, scientists have proposed that the inner core actually consists of two layers, referred to as the inner core and the innermost inner core.
Now, in a paper published in the journal Nature Communications Tuesday (Feb. 21), researchers looked at earthquake, or seismic, wave data from all over the world to measure this innermost inner core.
When an earthquake strikes, it triggers waves of energy that move through rock. These waves move at different speeds based on the kinds of minerals the rock is made of and whether the rock is more rigid or soft. Certain kinds of seismic waves can't move through liquid, so they bounce off a liquid layer. Studying the way seismic waves move through Earth can reveal what distinct layers exist deep below the planet's surface.
For example, scientists have previously used seismic waves to discover the churning, liquid iron of Earth's outer core, which creates the planet's magnetic field. Seismic waves have also revealed the inner core, which, despite the heat, remains solid under immense pressure.
In the new paper, the researchers "'observed, for the first time, seismic waves bouncing back and forth from a powerful earthquake to the other side of the globe, like ping-pong balls," study's lead author Thanh-Son Pham, a geophysicist at the Australian National University in Canberra, told Live Science in an email.
In particular, seismic waves from a magnitude 7.9 earthquake that struck near the Solomon Islands in 2017 reverberated across Earth's entire diameter several times. Seismic networks in the Alaskan Peninsula and European Alps helped the researchers see the reverberating waves, and these bouncing waves enabled the researchers to observe the two distinct layers within Earth's inner core.
Get the world’s most fascinating discoveries delivered straight to your inbox.
The researchers noted that when the earthquake waves traveled through the innermost core, in an area about 450 miles across, they moved at different speeds depending on the angle at which they were traveling. In the outer layer of the inner core, the waves moved the fastest from pole to pole and the slowest in an equatorial direction. In the innermost layer, meanwhile, the waves moved the slowest at an angle about 50 degrees away from Earth's axis.
The different behavior of the waves moving through the outer layer of the inner core versus the innermost inner core suggests that although they may be chemically identical (made of an iron-nickel alloy), the crystal structures of these layers are different, Pham said.
"This study strengthens the evidence for the existence of an internal metallic ball with a distinct texture from the outer shell of Earth's inner core," Pham said.
Earth's structure evolved as it cooled after the planet formed around 4.6 billion years ago. As Earth cooled, heavier elements, like iron and nickel, migrated inward, creating the inner and outer cores, while lighter elements — like the silicon that makes up much of the rock at Earth's surface — rose.
The new view of Earth's innermost inner core could suggest that an event early in the planet's history affected its formation, and that idea could change what we know about when and how the inner core formed, Pham said.
However, there's currently no way to know what kind of event could have created the distinct layer within the inner core, or when, Pham said.. Scientists think that Earth’s core formed about a billion years ago, but the details of the core’s evolution are not well understood. So it’s difficult to say when an event might have occurred that altered the innermost core. But as the global network of seismometers grows, more seismic data will likely help uncover more details about the inner core’s growth.
"The exact timeline of the possible global event is wildly uncertain," Pham said. "Answering those questions could go a long way in understanding the Earth's evolution."
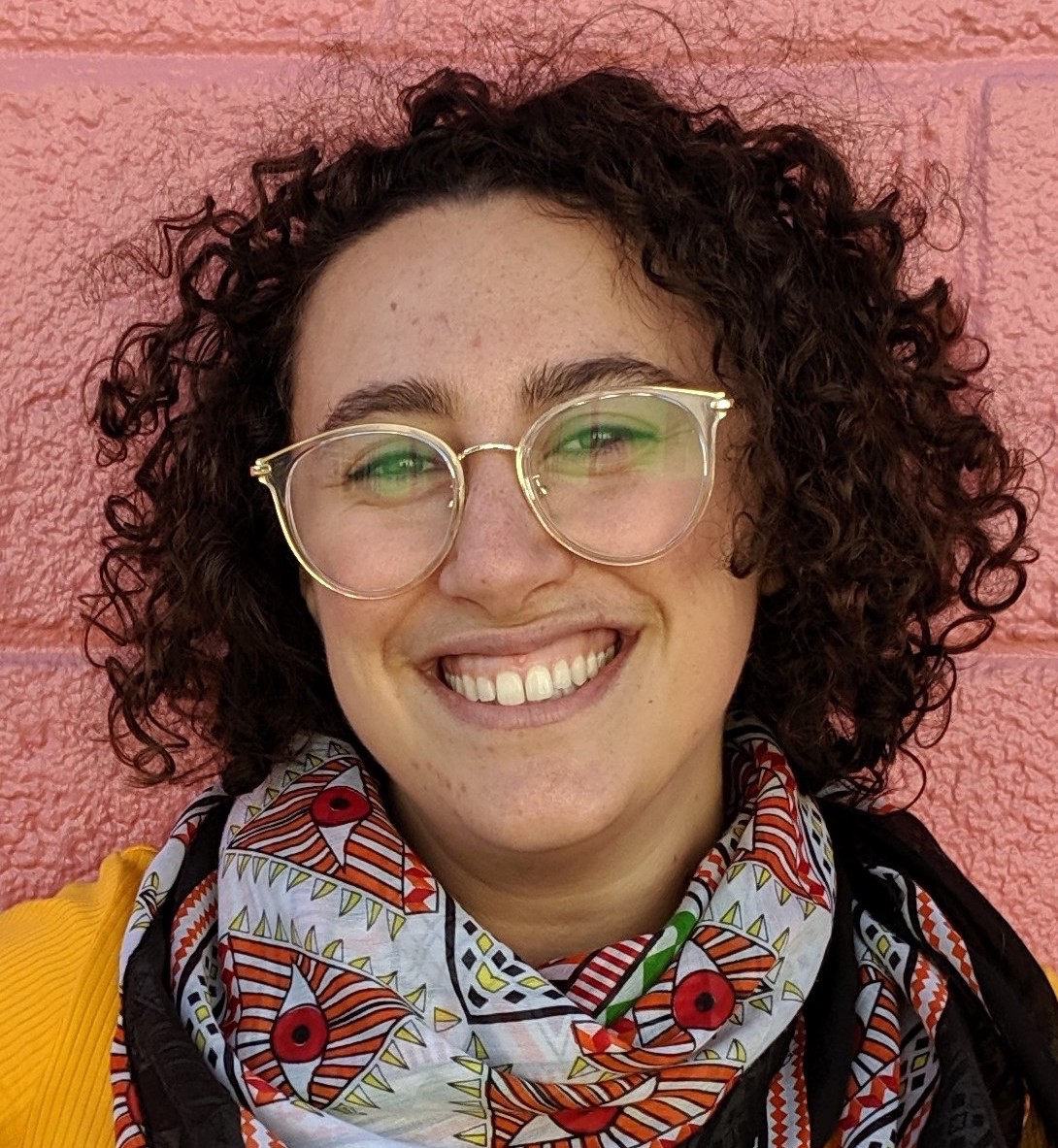
JoAnna Wendel is a freelance science writer living in Portland, Oregon. She mainly covers Earth and planetary science but also loves the ocean, invertebrates, lichen and moss. JoAnna's work has appeared in Eos, Smithsonian Magazine, Knowable Magazine, Popular Science and more. JoAnna is also a science cartoonist and has published comics with Gizmodo, NASA, Science News for Students and more. She graduated from the University of Oregon with a degree in general sciences because she couldn't decide on her favorite area of science. In her spare time, JoAnna likes to hike, read, paint, do crossword puzzles and hang out with her cat, Pancake.