Nanoscale Sensor Probes Cell’s Pulse
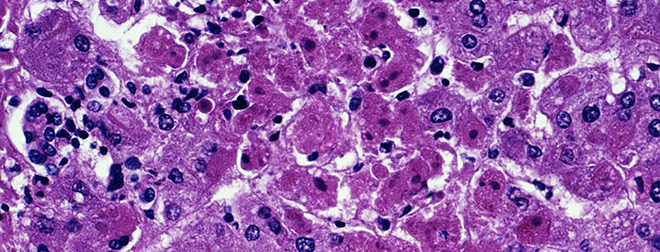
(ISNS) -- A heartbeat, or a pulse, is a standard indicator of whether a person or any other animal is dead or alive. But for a single biological cell, checking its viability – its state of being "alive" – is not that simple.
Knowing a cell's viability is valuable information in studying how an antibiotic works, how toxic substances kill cells and how an infection spreads. However, most viability tests for cells involve invasive procedures – such as injecting a dye into the cell. The tests are expensive and afterward, scientists have to dispose of the cells.
But now, a group of South Korean researchers have developed a non-invasive device that uses the cell's heat conductivity – its ability to act as a conduit for heat – to determine its viability.
Size does matter
A living cell is like an around-the-clock factory – food is constantly broken down, complex materials are continuously assembled and proteins move in and out. All of these activities either take in or give off heat. Tracking heat's passage through the cell could indicate how active the cell is, or if it is alive at all.
However, there is one tiny problem – the cell's size.
A single cell is a million times smaller in volume than a drop of water. At that scale, most devices cannot easily separate the cell's heat properties from surrounding sources of heat, according to Dongsik Kim from the Pohang University of Science and Technology in South Korea, senior author of the study.
Get the world’s most fascinating discoveries delivered straight to your inbox.
The researchers, however, solved this problem by creating an extremely tiny device which uses a common physical technique called the 3-omega method to measure the cell's heat conductivity value – known as its k value. They found that the higher the cell's k value, the less likely the cell is viable.
When a cell is alive, its outer wall tightly controls the movement of materials that carry heat in or out, thus maintaining a steady heat balance. When a cell dies, however, the outer walls start crumbling, letting water and other materials flow freely in and out of the cell, disrupting its inner heat "network."
Although a far cry from a Star Trek-esque bioscanner, the newly developed device was able to distinguish the k values of three different types of human and rat cells taken from the liver, a connective tissue and a lab-grown cell line.
The researchers found that dead cells from the liver and lab-grown samples had 6 and 13 percent higher k values, respectively, than living cells.
The researchers expect that the same principle could distinguish healthy cells from diseased cells. They also believe that measuring k values in individual cells can help determine the type of cell in the sample.
How it works
The device has a thin metal strip which is cast on a glass layer and touches a tiny well holding the cell sample. At 200 nanometers wide, the metal strip is about 400 times thinner than a strand of human hair.
In the 3-omega method, the metal strip is "activated" by an electric pulse and releases heat, which is picked up by the cell. How much heat the cell picks up depends on its k value. The transfer of heat creates a change in the output voltage which is used to calculate the cell's k value.
By doubling as the heat source and a sort of gauge, the metal strip eliminates the need for bulky instruments, thereby greatly reducing the size of the device. Its miniscule size also ensures that the heat it generates is passed on to the cell sample alone and not to the cell’s surroundings, Kim said.
The new device shows potential for testing inside the human or animal body, in principle, he said.
"One of the future applications of the technology is to install the sensor at the tip of an endoscope. Then, without taking a cell sample out, we may analyze the … tissue," he wrote in an email.
The 3-omega method has been used for decades to study the heat properties of a wide range of materials from light bulbs to carbon nanotubes. Kim and his colleagues have been working on the technique for many years and succeeded in analyzing tiny liquid samples only recently.
What’s innovative, however, is applying this measurement tool to a new system, a biological system, said Jonathan Malen, a mechanical engineer at Carnegie Mellon University in Pittsburgh.
"It is very interesting how they’ve adapted the 3-omega method to measure the conductivity of a single cell," Malen said.
The research was published in Applied Physics Letters.
Inside Science News Service is supported by the American Institute of Physics. Ranjini Raghunath is a science writer in Mountain View, California.