The Right and Wrong Moves of Cell Migration
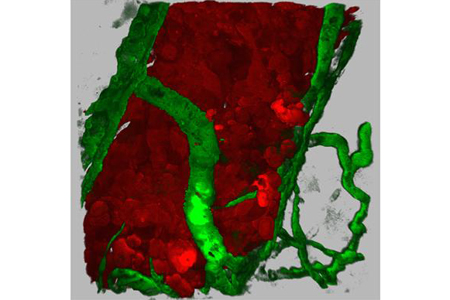
Birds do it, butterflies do it — but did you know that cells in our bodies do it, too? Migrate, that is.
Cells move from one location to another to help keep us alive and well.
If cell migration veers off course, serious health problems can result, including cancer, birth defects and chronic inflammation, such as in arthritis. Scientists funded by the National Institutes of Health are studying cell migration with the goal of developing better treatments for these and other conditions.
Here are three examples of how cells move in ways that harm or help us.
Malevolent Migrants
The specific details of how a cell gets from point A to point B vary depending on the cell type and the circumstances. While some cells crawl at a stately pace, others dash. Some journey as loners; others travel in packs. Tumor cells, for instance, can migrate individually or in small groups. As the result of various genetic mutations, they can acquire the ability to invade surrounding tissues and move long distances through the blood or lymph vessels to take up residence in distant locations in the body. This process, known as metastasis, is a major cause of complications and death in people with cancer.
Richard Klemke and his colleagues at the University of California, San Diego School of Medicine, are using zebrafish to study how tumor cells spread. By injecting the tiny, transparent fish with human breast cancer cells, the researchers can watch tumor cells invade and move through the walls of nearby blood vessels — a key step in cancer metastasis. The technique has helped them identify proteins needed for cancer cells to enter the bloodstream. Because cells in zebrafish and many other organisms, including us, use the same basic processes to get around, these findings may aid the search for medicines that slow or stop the spread of cancer in humans.
Sign up for the Live Science daily newsletter now
Get the world’s most fascinating discoveries delivered straight to your inbox.
Dance of Development
Like choreographed dancers, cells move in a coordinated manner to help form tissues, organs and limbs during embryonic development. The carefully timed migration of groups of embryonic stem cells produces distinct layers of the embryo that are further shaped and folded into structures such as the neural tube, which gives rise to the spinal cord and brain. Defective cell migration during development can lead to a wide range of birth defects that cause physical, intellectual or developmental disability and other health problems.
To better understand how our cells get moving during development, scientists have turned to another transparent organism, C. elegans. This tiny roundworm can grow from a single fertilized egg to a fully developed and functioning organism in just 14 hours. These and other features — including its many genes and cellular processes similar to ours — make the worm ideal for studying development.
In one stage of development, certain cells migrate from the embryo's surface to its interior to form internal structures. Scientists used to think this migration began as soon as a "motor protein" called myosin started up and tugged on a mesh of filaments just under the cell surface, causing the surface to shrink and pinch inward. But research in the roundworms indicates that firing up myosin isn't enough to start cell pinching.
Findings from Bob Goldstein's lab at the University of North Carolina, Chapel Hill, suggest that cells, like cars, engage a clutch-like mechanism that couples the myosin engine with the cell surface to drive pinching. Learning how this molecular clutch works could help lay a foundation for the future diagnosis and prevention of spina bifida and other neural tube defects, which are among the most common and serious human birth defects.
Double-Edged Sword of Inflammation
White blood cells serve many functions, but their primary job is to help the immune system protect the body from infection. Therefore, they need to move quickly to an injury or infection site. The pain of inflammation, such as that caused by a sprained ankle or a cut on the skin, is a consequence of hordes of white blood cells rushing into injured tissue and spilling noxious chemicals that help fight foreign invaders. When inflammation becomes chronic, diseases such as asthma or arthritis can result. In rheumatoid arthritis, for example, abnormal migratory signals cause certain white blood cells to home to the joints. The immune cells damage supporting tissue, leading to persistent pain and inflammation.
Infection-fighting white blood cells called neutrophils and primitive single-celled organisms such as amoebae have an internal "compass" that lets them sense and move toward chemicals in the environment. Through this process, known as chemotaxis, neutrophils pursue an invader and amoebae inch toward a food source.
Peter Devreotes of the Johns Hopkins University School of Medicine studies chemotaxis in a bacteria-eating amoeba named Dictyostelium that lends itself to powerful genetic analysis. By studying amoebae with genetic defects affecting chemotaxis, Devreotes has uncovered biochemical communication systems within the amoebae that are important for chemotaxis and shown that some of these systems also guide migration in neutrophils and many other types of cells.
If scientists can learn how our cells know where to migrate and what makes them move, it may open paths to controlling cell behavior, improving the treatment of chronic inflammation and preventing cancer metastasis. Detailed insights on cell movement could also lead to new drugs to promote wound healing and even to ways to grow new tissues and organs from scratch.
This Inside Life Science article was provided to LiveScience in cooperation with the National Institute of General Medical Sciences, part of the National Institutes of Health.
Learn more:
Also in this series: