How to Listen to One Brain Cell at a Time
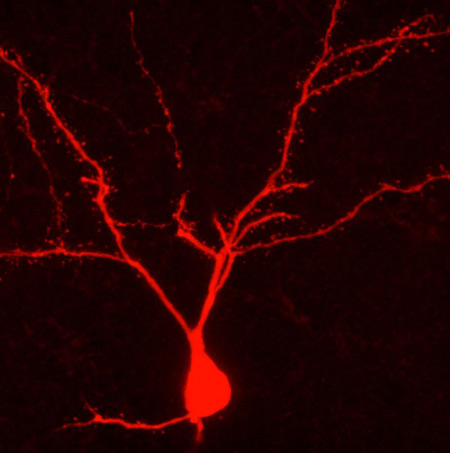
This Behind the Scenes article was provided to LiveScience in partnership with the National Science Foundation. Carl Schoonover is an NSF Graduate Research Fellow and a neuroscience doctoral candidate at Columbia University, where he studies the physiology and anatomy of single neurons in the rodent brain. He is also a member of the Columbia Neuroscience Writing group (neuwrite.org) and his book "Mind in Sight," will be published in Fall 2010. To measure the activity of neurons in anesthetized rodent brains and mark them for anatomical study, Schoonover uses a method called "juxtacellular recording" that was invented by French neuroscientist Didier Pinault. Pinault described the method in a 1996 paper published in the Journal of Neuroscience Methods with the lengthy title, "A novel single-cell staining protocol performed in vivo under electrophysiological control: morpho-functional features of juxtacellularly labeled thalamic cells and other central neurons with biocytin or Neurobiotin." As is often the case, Pinault’s description of the method (in boldface below) is geared towards scientists. In describing his own work, Schoonover translates the text of his field for a broad audience, conveying some of the intangibles inherent to the process. Pinault: Micro-electrodes were pulled from 2 millimeter glass capillaries containing a micro-filament to an external diameter ranging from 1.0 to 1.3 micrometer, and were filled with solution containing the marker molecules. Schoonover: There is an instrument in my lab, the DMZ-Universal Puller, that performs feats with glass that surpass those of even the most virtuosic Venetian glassblowers. Each of its micro-electrode creations is unique, even if their distinctive marks are apparent only to the appreciative eye of the most committed admirers. The machine is no doubt aware of its caliber, and so is unpredictably sensitive, temperamental even; there is no telling how the time of day, the vagaries of the weather or the intricacies of the t(F1) setting may bear upon its mood and art. We followers are grateful for its masterpieces and bite our tongues when the work disappoints. Regardless, we scrutinize everything, study and opus, under the lens of a 100X magnification microscope in search of the perfect micro-electrode tip. Each of us, the apprentices, must place a hollow cylinder of glass approximately 2 millimeters in diameter and over 50 in length, into the master's confident grasp. The DMZ-Universal Puller envelops the middle of the cylinder with a hot filament, and with a quick, precise motion and a satisfying click, it begins to tug the glass from either end. The glass, enveloped by the burning filament, begins to relax, melt, lose its identity — and stretch, like a string of pink bubble gum caught between the teeth and fingers of a cheeky 14-year-old. A moment later, the entire apparatus jolts, the glass snaps and one cylinder becomes two. Where the heat had been applied there are now two opposing cones of cooling glass, and at the end of each one of them, a hollow tip, now one thousandth of a millimeter in diameter. The ideal shape of the micro-electrode is as immediately recognizable as it is difficult to describe. At the tip, the microscopic hole at the end of the glass cone, we seek perfect symmetry, a miniscule opening neither too large nor too small, its edge never round enough, and lips, generous, lavish lips, for soon it must delicately approach and kiss its mate. I know what I mean. Pinault: Connected to an intracellular recording amplifier, such a micro-pipette (DC resistance: 5-7 mega-Ohm), was moved down with a stepping micro-driver. Schoonover: The micro-electrode tip is the oracle through which neurons and humans converse. I fill my electrode with a solution into which I dip a fragment of wire, itself connected to an amplifier. What the tip hears, my amplifier now hears; our common language is Ohm's law. I clasp the electrode onto a third instrument —precise, reliable, utterly charmless. It ushers the tip of my electrode to the exposed surface of a rat's brain and with microscopic precision guides it into its depths. Pinault: The background noise usually increased just before reaching a juxtacellular position. Schoonover: As the tip descends through the cortical layers of the brain it registers continuous waves of sound, thousands of distant neural murmurs of which I can make no sense. There is a universe in here. My ear straining for clues, I await the moment when the sound grows louder, surges, as if the volume were raised on a television whose broadcast has long ceased. A groundswell of aural snow. Ohm's Law would have it that when glass encounters biological membrane, the resistance between the wire fragment and the ground wire jumps, and so the noises around us are amplified. Now there is a blizzard in the lab. I have found what I am looking for. Pinault: Identification of the target neurons was usually achieved through knowledge of their electrophysiological and synaptic properties ... Once a cell was juxtacellularly recorded, well isolated, and identified, biocytin was applied under electrophysiological control to ensure that the recorded neuron remained alive. Employing the bridge circuitry of the recording amplifier, the histochemical tracer was first applied with a 50% duty cycle of 200 milliseconds anodal current pulses of increasing intensity (usually <10 nano-Amperes). Schoonover: The murmurs become inaudible when I set my amplifier to filter out all slow sounds and focus on the fast; fast, like the events that register as isolated popcorn explosions on my speakers now. Each time, an action potential materializes on my oscilloscope screen and commands the same fascination from me as the canonical "spike" did, decades ago, when it leapt out of a squid axon and into the history of science. Each spike begins with a wave of electrical current flowing into the cell, shooting the green line on my oscilloscope upward, only for it to fall a moment later as current gushes out of the cell with the same haste. The action potential, the currency of the brain, looks like an upside-down V. The eye takes over where the ear left off, and I turn down the audio speakers to concentrate on the green trace continuously updating before me, like an EKG. At first each cell appears the same as all the others I've seen before, but soon each one's personality begins to emerge. My role is to bear witness to it. Some are chatty, delivering their soliloquy in bursts as if too excited to take the time for every syllable; others are more guarded, almost hesitant, or perhaps they are just above the fray. And the exact shape of each cell’s spikes — oh, it is an entire subgenre of its own. Words like "fat," "slim," "long," "tall," "short," "loud," "quiet," "fast" and "slow" litter the margins of my notebooks as each one attains an immortality of sorts. This subjective minutiae is the stuff that science is made of. There is only so much that I can learn from eavesdropping, no matter how carefully. The patchwork of inferences that I am allowed to draw from my experiment is that much richer if I know the exact position of the cell I've just recorded, or am granted detailed knowledge its shape. For in the world of neurons, shape is everything: protuberances called axons and dendrites shoot out of its center, and travel sometimes millimeters in distance — the information highways of the brain. If I know what a neuron looks like, I can tell you how it fits into this network, and who it’s been talking to. And so in the solution that fills the micro-electrode I have included a chemical spy; the trick is to sneak it into the meticulously maintained space on the other side of the membrane by delicately disrupting it. Once the spy is in I can illuminate the cell from the inside. For all its coarseness, the Pulser comes in handy at this stage. Its centerpiece is a black knob the size of a thumbnail that determines the magnitude of the electrical current that I am about to deliver to the very end of the electrode's tip. I program the Pulser to switch on for 200 milliseconds, and then off for that same amount of time, and I carefully — fearfully turn the knob to the right, increasing the electrical shocks one tick at a time, and lean into the oscilloscope. Pinault: From this critical moment, pulse intensity was adjusted (usually between 1 and 5 nano-Amperes) such as to prevent obvious cellular damage, the first hallmark being action potential broadening which could be preceded by a transient DC shift of about -40 millivolts. Schoonover: As the membrane is punctured by the electrical pulses through the tip, my electrode, my amplifier, and I gain access to the cell's inner sanctum and deliver current directly inside it — my chemical spy along with it — causing the cell to discharge frenetic spikes in response. The tense minutes that follow are a balancing act between disrupting the cell just enough and overwhelming it altogether. It is undoubtedly a sorry sight, that of the graduate student hunched over a metal box late at night, tensely gripping a small, stubborn knob in his sweaty fingers as he reacts to the mood of a patch of membrane a couple microns in area. At this critical stage, I must adjust the current intensity at every instant in order to avoid electrocuting it into oblivion. The danger is a death fit for the opera stage, a sequence of spikes that fly out of control, as the cell's entire membrane disintegrates under the shock, and the world outside starts seeping in. First a woodpecker, then a machine gun, then, as the spikes become impossible to distinguish, an existential wail whose pitch steadily descends until nothing remains but silence and a flat line on the oscilloscope. Sometimes, as if aware of the effect of its dramatic genius on its spellbound audience, a cell will emerge in a Violetta moment, for one last gasp. Pinault: During each attempt at filling a cell juxtamembranously its electrical behavior usually oscillated in a rhythmic manner, in phase with the current pulses. In other words, juxtacellular application of tracer molecules thus consisted of "tickling" the investigated cell as long as necessary (for a few seconds up to 26 minutes in this study). Schoonover: It is easy enough to rationalize; there are millions more healthy cells just like it in the anesthetized rat I am studying: this sort of cataclysm affects only an infinitesimally small part of its brain. Nevertheless, the loss is devastating every time. I have taken to cutting the audio feed if things begin to head south. But in the best of times, my cell dutifully fires spikes in phase with the Pulser's onset every half-second, and keeps silent when it is off, a perfect balance. It is critical, after a few minutes of this treatment, to allow it to recover from the affront, a task I find particularly haunting. To give the cell the privacy it needs to pull itself together, I walk over to the other side of the other room keeping an ear open and reading Maureen Dowd, trying not to worry. The trick is to pretend like I just don't care. Editor's Note: This research was supported by the National Science Foundation (NSF), the federal agency charged with funding basic research and education across all fields of science and engineering. See the Behind the Scenes Archive.
Sign up for the Live Science daily newsletter now
Get the world’s most fascinating discoveries delivered straight to your inbox.