'Smart' Hands Hold Promise for Amputees
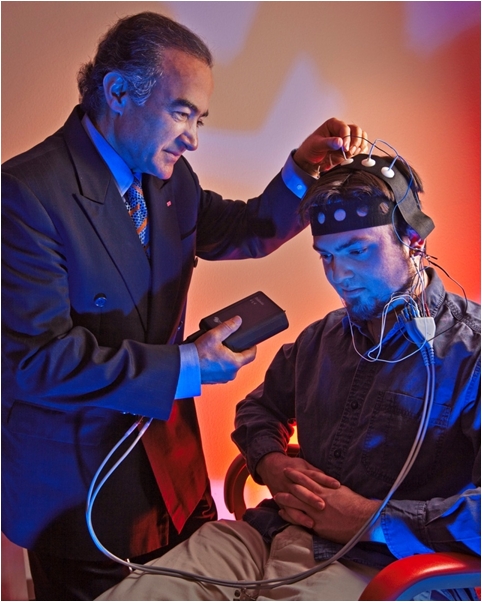
This Research in Action article was provided to LiveScience in partnership with the National Science Foundation.
Even though our hands are not a vital organ, can you imagine having to live without them?
Prosthetic limbs made with wood and many other materials have been used for centuries, with both aesthetic and functional purposes.
Today's engineers and scientists are working to produce prosthetic limbs as similar as possible to the real ones.
To be able to move our hands, first we need to generate a stimulus in the part of our brain called the motor cortex. The stimulus travels to the spinal cord, continues through the nerves, and ultimately reaches the muscles that need to be activated to be able to open, close or make any other movements with our hands.
To be able to feel something with our hands, the process is reversed. Special receptors in the skin are stimulated by temperature, pressure or pain. The stimulus travels through the nerves to the spinal cord and then to the brain (to the sensory cortex), where the message is interpreted.
The image above reveals a non-invasive direct brain control technique for limbs and brain-intention detection. There are several techniques that allow reading the electrical stimuli that are produced in the brain:
Sign up for the Live Science daily newsletter now
Get the world’s most fascinating discoveries delivered straight to your inbox.
- Electroencephalography (EEG), which has been used for many years in the diagnosis of epilepsy. This uses non-invasive electrodes on the scalp to record the electrical activity from the brain. This technique is inexpensive and does not have medical complications, but it is imprecise.
- Electrocorticography (ECoG), in which the electrodes are placed on the brain. The invasive technique allows a better reading, but may have more medical complications such as infection or bleeding.
- Magnetoencephalography (MEG), which reads magnetic fields produced by the electrical stimuli, is non-invasive, but expensive.
- Functional Near Infrared (FNIR) Imaging, which identifies the parts of the brain producing the stimuli by the increase in the amount of blood in that area based on infrared radiation measurement, is non-invasive and inexpensive.
Researchers have now created a prosthetic hand that can feel and can reproduce many of the movements of the human hand. That type of prosthesis works with a chip that is implanted on the brain and which reads the stimuli from the motor cortex, transmitting and receiving signals to and from the prosthesis.
The signals do not need to go to the spinal cord; they can go straight from the brain to the machine, a system known as a brain-computer interface (BCI). Such an interface is very important when dealing with patients who have damage in the spinal cord and are not able to transmit signals through it.
One of the main problems with the chip is that it disintegrates over time from contact with human tissues and needs to be replaced every couple years, which increases the risk of infection and bleeding.
Rahmat Shoureshi, at the University of Denver, is working on a prosthesis that uses combined imaging techniques to read the stimuli from the brain.
"Both fNIR and EEG are used in our hybrid sensory system, and combined they have a superior ability to discern brain intentions compared to EEG alone," said Shoureshi. "While technologies such as fMRI and MEG may have higher sensitivity and increased resolution, they are expensive and require large equipment. EEG and fNIR are both low cost and portable systems suitable for real-time brain monitoring."
While unable to relay sensory feedback to the user, the non-invasive nature is an advantage. The technology is safer than invasive systems, yet can accurately read the activity of the neurons in the motor cortex and avoids interference (which would result in imprecise movements).
"The resolution of the fNIR imaging system is only limited by the spacing of the light emitting and receiving elements," said Shoureshi. "In our current design, the spacing between source and sensor is one inch, which we have found sufficient for our current use of the technology. If a higher resolution fNIR is necessary, we have already built and tested systems that overlap source and sensor groups."
Any opinions, findings, and conclusions or recommendations expressed in this material are those of the author and do not necessarily reflect the views of the National Science Foundation. See the Research in Action archive.