Exploring the Elusive World of Life's Most Vital Proteins
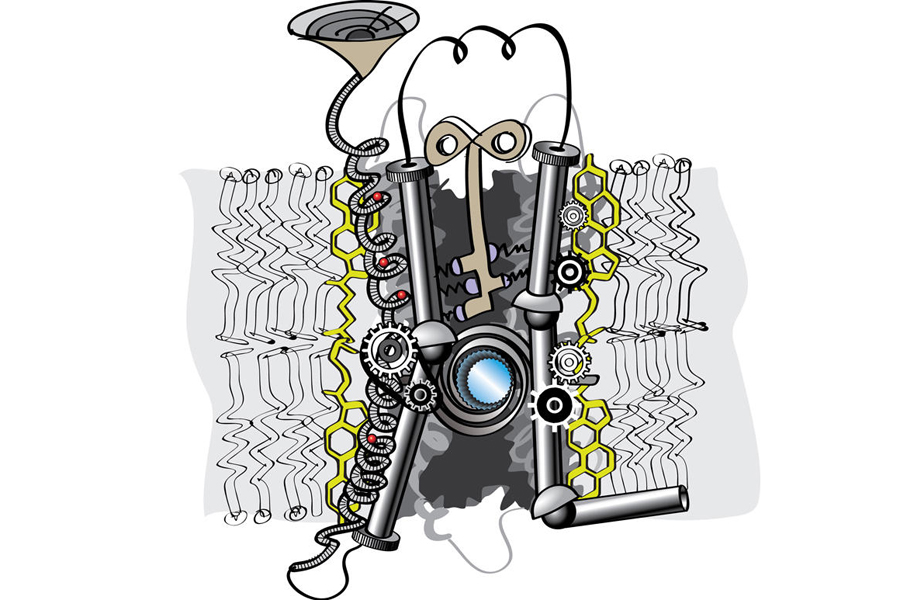
GPCR — that's an abbreviation you may have heard only recently, when the 2012 Nobel Prize in chemistry recognized groundbreaking work on this important family of proteins.
These proteins, G protein-coupled receptors, control practically every bodily process. Scientists estimate that there about 800 different types in the membranes of your cells. Some are sensing molecules that let you see, smell and taste; others give you a boost after a few sips of coffee, make you retreat during a conflict or help fight off infection. GPCRs also are associated with diseases ranging from asthma to schizophrenia, and they are thetarget of more than a third of marketed drugs, including allergy and heart medications and antidepressants.
Yet GPCRs' structures — key to understanding how they work and to designing more effective drugs — have remained relatively hidden from view. Researchers funded by the National Institutes of Health have given us a peek at some of them, and the improved methods these scientists have developed could reveal lots more structures in the near future.
Structural Clues
Labs around the world have tried for years to obtain detailed images of human GPCRs because the precise, three-dimensional arrangement of a protein's atoms provides important details about how a protein interacts with its natural partner molecules in the body or with drug molecules. But the structures of membrane proteins, including GPCRs, are as difficult to determine as they are valuable to understand.
One challenge is that GPCRs are exceedingly flimsy and fragile when not anchored within their native cell membranes. This makes it very hard to coax them to form crystals so that their structures can be determined through X-ray crystallography.
Right now, we know the structures of about 1 percent of all human GPCRs, and researchers are using two key approaches to generate and study more. Stanford University's Brian Kobilka, who shared the 2012 Nobel Prize for his work on GPCRs, is among the scientists who are focusing on specific GPCRs to better understand how they function and interact with other molecules, including drugs. Other scientists, such as Raymond Stevens at The Scripps Research Institute, are taking a complementary approach to get structures that represent each of the major branches of the GPCR family tree. Knowing more about one member could enable scientists to computationally model the others.
Sign up for the Live Science daily newsletter now
Get the world’s most fascinating discoveries delivered straight to your inbox.
GPCR Gallery
Here are some of the key structures that the Stevens group and its collaborators, including Kobilka and other scientists around the world, have solved using the family tree approach:
- The first high-resolution structure of a human GPCR, the molecular "fight or flight" switch called the β2 adrenergic receptor.
- The A2A adenosine receptor, sometimes called the "caffeine receptor." Our bodies sense and respond to caffeine the same way they do to fragrances, light and other stimuli. Tweaks to this GPCR molecule make it send a signal from the cell's external environment to its interior.
- The CXCR4 chemokine receptor, which normally helps activate the immune system and stimulate cell movement. But when the signals that activate the receptor aren't properly regulated, CXCR4 can spur the growth and spread of cancer cells. To date, CXCR4 has been linked to more than 20 types of cancer.
- The D3 dopamine receptor, which plays a vital role in the central nervous system, affecting our movement, cognition and emotion.
- The H1 histamine receptor, which plays a role in how the immune system produces allergic reactions to pollen, food and pets. Many allergy medications work by blocking the action of this type of GPCR.
- The kappa opioid receptor, a protein on the surface of brain cells that is centrally involved in pleasure as well as in pain, addiction, depression, psychosis and related conditions. Dozens of legal and illegal drugs, from anesthetics to heroin, work by targeting these receptors.
Technical Breakthroughs
What was the game-changing technical breakthrough that has made determining these structures possible?
"I'm always asked that question," says Stevens, "and the answer is that there wasn't just one breakthrough, there were about 15 separate developments by several different investigators around the world, each breakthrough critically needed in combination with one another, and they came together after a long time."
Some of these breakthroughs have improved researchers' ability to produce and purify GPCRs in quantities sufficient for crystallization.Other breakthroughs have been aimed at stabilizing GPCRs, making them more crystallizable and holding them in a specific structural conformation. Scientists continue to improve other methods, including the ability to model new GPCR structures from known ones.
These developments have had an enormous impact on furthering our understanding of GPCRs, and they should lead to new insights on biological processes and aid progress in drug discovery.
Learn more:
Video of HIV Viral Protein Binding to CXC4 Receptor
GPCR Dock 2013 Modeling Competition
Also in this series:
Studying Protein Shapes Helps Combat HIV
This Inside Life Science article was provided to LiveScience in cooperation with the National Institute of General Medical Sciences, part of the National Institutes of Health.