We may finally know what life on Earth breathed before there was oxygen
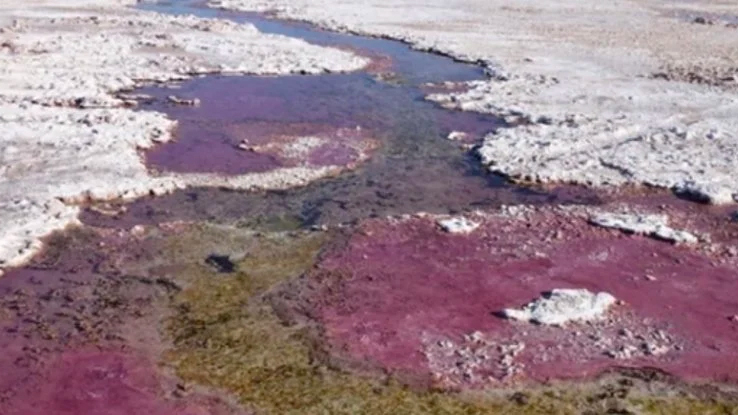
Billions of years ago, long before oxygen was readily available, the notorious poison arsenic could have been the compound that breathed new life into our planet.
In Chile's Atacama Desert, in a place called Laguna La Brava, scientists have been studying a purple ribbon of photosynthetic microbes living in a hypersaline lake that's permanently free of oxygen.
"I have been working with microbial mats for about 35 years or so," says geoscientist Pieter Visscher from the University of Connecticut.
"This is the only system on Earth where I could find a microbial mat that worked absolutely in the absence of oxygen."
Microbial mats, which fossilize into stromatolites, have been abundant on Earth for at least 3.5 billion years, and yet for the first billion years of their existence, there was no oxygen for photosynthesis.
How these life forms survived in such extreme conditions is still unknown, but examining stromatolites and extremophiles living today, researchers have figured out a handful of possibilities.
While iron, sulphur, and hydrogen have long been proposed as possible replacements for oxygen, it wasn't until the discovery of 'arsenotrophy' in California's hypersaline Searles Lake and Mono Lake that arsenic also became a contender.
Sign up for the Live Science daily newsletter now
Get the world’s most fascinating discoveries delivered straight to your inbox.
Since then, stromatolites from the Tumbiana Formation in Western Australia have revealed that trapping light and arsenic was once a valid mode of photosynthesis in the Precambrian. The same couldn't be said of iron or sulphur.
Just last year, researchers discovered an abundant life form in the Pacific Ocean that also breathes arsenic.
Even the La Brava life forms closely resemble a purple sulphur bacterium called Ectothiorhodospira sp., which was recently found in an arsenic-rich lake in Nevada and which appears to photosynthesize by oxidising the compound arsenite into a different form -arsenate.
While more research needs to verify whether the La Brava microbes also metabolize arsenite, initial research found the rushing water surrounding these mats is heavily laden with hydrogen sulphide and arsenic.
If the authors are right and the La Brava microbes are indeed 'breathing' arsenic, these life forms would be the first to do so in a permanently and completely oxygen-free microbial mat, similar to what we would expect in Precambrian environments.
As such, its mats are a great model for understanding some of the possible earliest life forms on our planet.
While genomic research suggests the La Brava mats have the tools to metabolize arsenic and sulphur, the authors say its arsenate reduction appears to be more effective than its sulfate reduction.
Regardless, they say there's strong evidence that both pathways exist, and these would have been enough to support extensive microbial mats in the early days of life on Earth.
If the team is right, then we might need to expand our search for life forms elsewhere.
"In looking for evidence of life on Mars, [scientists] will be looking at iron and probably they should be looking at arsenic also," says Visscher.
It really is so much more than just a poison.
The study was published in Communications Earth and Environment.
This article was originally published by ScienceAlert. Read the original article here.
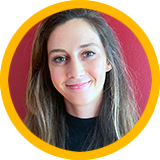
Carly Cassella is a journalist at ScienceAlert with a background in neuroscience. Carly cut her journalistic teeth at Farrago magazine while studying as an undergraduate at the University of Melbourne. Previously, she worked at the International Federation of Journalists in Brussels, where she gained the utmost respect for war correspondents. Since then, she has worked in award-winning podcast production, taught a class on science writing at the 2018 March for Science conference, and has written multiple YouTube scripts with millions of views. Carly currently lives in Seattle, where she enjoys clamming, oystering, fern-ing and pretending she knows how to identify birds and stars.