
First-Ever Observation of Higgs Boson Decay Opens New Doors for Particle Physics
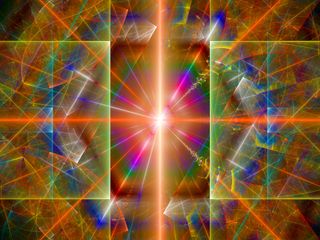
If you’ve been a science fan for the last few years, you’re aware of the exciting results to emerge from the Large Hadron Collider (LHC), which in 2012 found the Higgs boson, the subatomic particle responsible for giving mass to fundamental subatomic particles.
Today, physicists have another exciting announcement to add to the Higgs saga: They have made the first unambiguous observation of Higgs bosons decaying into a matter-antimatter pair of bottom quarks. Surprisingly, the Higgs bosons decay most often in this way.
The new announcement shows a strong agreement between the theoretical predictions and the experimental data, which could in turn set strict constraints on ideas of more fundamental physics that strive to explain why the Higgs boson even exists.
Field of dreams
In the 1960s, researchers were investigating linkages between the force of electromagnetism and the weak nuclear force, which is responsible for some types of radioactive decays. Although the two forces seemed distinct, it turned out that they both arose from a common and more fundamental force, now called the electroweak force.
However, there was a problem. The simplest manifestation of the theory predicted that all particles had zero mass. Even in the 1960s, physicists knew that subatomic particles had mass, so that was potentially a fatal flaw.
Several groups of scientists proposed a solution to this problem: A field permeates the universe, and it's called the Higgs field. Fundamental subatomic particles interacted with this field, and this interaction gave them their mass. [6 Implications of Finding the Higgs Boson]
The existence of the field also implied the existence of a subatomic particle, called the Higgs boson, which was finally discovered in 2012 by researchers working at the European Organization for Nuclear Research(CERN) laboratory in Switzerland. (Disclosure: I am a collaborator on one of the research groups that made the initial discovery as well as today’s announcement.) For their predictions of the Higgs field, British physicist Peter Higgs and Belgian physicist François Englert shared the 2013 Nobel Prize in physics.
Sign up for the Live Science daily newsletter now
Get the world’s most fascinating discoveries delivered straight to your inbox.
Finding the bottom quarks
Higgs bosons are made in high-energy collisions between pairs of particles that have been accelerated to nearly the speed of light. These bosons don’t live for very long — only about 10^minus 22 seconds. A particle with that lifetime, traveling at the speed of light, will decay long before it travels a distance the size of an atom. Thus, it is impossible to directly observe Higgs bosons. It is only possible to observe their decay products and use them to infer the properties of the parent boson.
Higgs bosons have a mass of 125 gigaelectron volts (GeV), or one that's about 133 times heavier than a proton. Calculations from well-established theory predicts thatHiggs bosonsdecay into pairs of the following particles in the following percentages: bottom quarks (58 percent), W bosons (21 percent), Z bosons (6 percent), tau leptons (2.6 percent) and photons (0.2 percent). More exotic configurations make up the remainder. One of the key results of today’s announcement was to verify that the prediction was correct for bottom quarks. [Strange Quarks and Muons, Oh My! Nature's Tiniest Particles Dissected] When physicists announced the discovery of the Higgs boson in 2012, they relied on its decay into Z bosons, W bosons and photons, but not bottom quarks. The reason is actually extremely simple: Those particular decays are far easier to identify. At the collision energies available at the LHC, Higgs bosons are made in only one collision in every 1 billion. The vast number of collisions at the LHC occur through the interaction of the strong nuclear force, which is (by far) the strongest of the subatomic forces and is responsible for holding the nucleus of atoms together.
The problem is that in interactions involving the strong force, production of a matter-antimatter pair of bottom quarks is really quite common. Thus, the production of bottom quarks by Higgs bosons decaying into bottom quarks is totally swamped by pairs of bottom quarks made by more ordinary processes. Accordingly, it is essentially impossible to identify those events in which bottom quarks are produced through the decay of Higgs bosons. It's like trying to find a single diamond in a 50-gallon drum full of cubic zirconia.
Because it is difficult or impossible to isolate collisions in which Higgs bosons decay into bottom quarks, scientists needed another approach. So, researchers looked for a different class of events — collisions in which a Higgs boson was produced at the same time as a W or Z boson. Researchers call this class of collisions "associated production."
W and Z bosons are responsible for causing the weak nuclear force and they can decay in distinct and easily identifiable ways. Associated production occurs less often than nonassociated Higgs production, but the presence of W or Z bosons greatly enhances the ability of researchers to identify events containing a Higgs boson. The technique of associated production of a Higgs boson was pioneered at the Fermi National Accelerator Laboratory, located just outside Chicago. Because of the facility's lower-energy particle accelerator, the laboratory was never able to claim that it had discovered the Higgs boson, but its researchers' knowledge played a significant role in today’s announcement.
The LHC accelerator hosts two large-particle physics detectors capable of observing Higgs bosons — the Compact Muon Solenoid (CMS) and A Toroidal LHC Apparatus (ATLAS). Today, both experimental collaborations announced the observation of the associated production of Higgs bosons, with the specific decay of Higgs bosons into a matter-antimatter pair of bottom quarks.
Theoretical Band-Aid
While the simple observation of this decay mode is a significant advance in scientific knowledge, it has a much more important result. It turns out that the Higgs field, proposed back in 1964, is not motivated by a more fundamental idea. It was simply added on to the Standard Model, which describes the behavior of subatomic particles, as something of a Band-Aid. (Before the Higgs field was proposed, the Standard Model predicted massless particles. After the Higgs field was included as an ad hoc addition to the Standard Model, particles now have mass.) Thus, it is very important to explore the predictions of decay probabilities to search for hints of a connection to an underlying theory. And there have been more recent and comprehensive theories developed since the 1960s, which predict that perhaps more than one type of Higgs boson exists.
Thus, it is crucial to understand the rate at which Higgs bosons decay into other particles and compare it with the predicted decay rates. The easiest way to illustrate agreement is to report the observed rate of decay, divided by the predicted rate. Better agreement between the two will yield a ratio close to 1. The CMS experiment finds excellent agreement in today’s announcement, with a ratio of predicted-to-observed rates of 1.04 plus or minus 0.20, and the ATLAS measurement is similar (1.01 plus or minus 0.20). This impressive agreement is a triumph of current theory, although it does not indicate a direction toward a more fundamental origin for the Higgs phenomena.
The LHC will continue to operate through early December. Then it will pause operations for two years for refurbishing and upgrades. In the Spring of 2021, it will resume operations with considerably enhanced capabilities. The accelerator and detectors are expected to continue to take data through the mid-2030s and to record over 30 times more data than what's been recorded so far. With that increase of data and improved capabilities, it is quite possible that the Higgs boson still has stories to tell.
Originally published on Live Science.
Don Lincoln contributed this article to Live Science's Expert Voices: Op-Ed & Insights.