
Last-Ditch Hack Led to the Invention of Quantum Mechanics
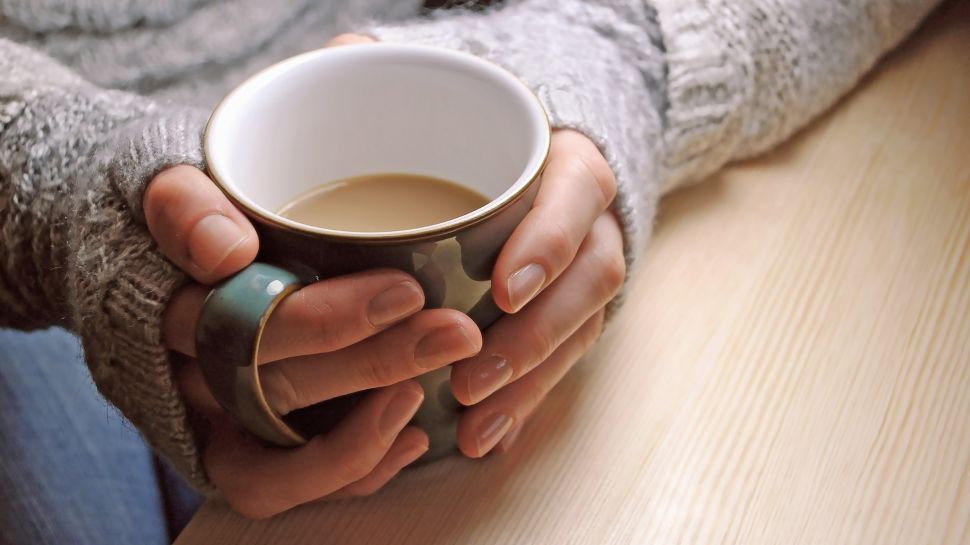
Let's say you've got some random object in front of you — how about a nice hot cup of coffee? You can see the cup and the coffee, of course, because light from your lamp is reflecting off it and into your eyeballs. And you can feel its warmth as you grab the mug. But hold your hand just beside the cup. You still feel a bit of warmth, don't you?
That's because the cup of coffee is indeed making its own kind of light, but it's not the visible kind. It's emitting light with a wavelength longer than the deepest red you could possibly imagine. What's below the red? Infrared. It may not be visible, but it's still a kind of light.
Related: Boom! Powerful Cosmic Explosion May Hint at How Black Holes Form
But your coffee cools while sitting on your desk, and eventually, you won't be able to feel the radiating warmth. Why did the hot cup of coffee emit radiation that you could feel, but the cold cup of coffee didn't? Who decides what kind of radiation is emitted when?
Black-box theater
This was a huge, burning question in the minds of 19th-century physicists, who had been studying a particular kind of radiation called blackbody radiation. The name comes from the experimental device used to investigate this phenomenon: a box coated in black on the interior, with a teensy-tiny pinhole for viewing.
A better name for this phenomenon is thermal radiation. It's the radiation given off by … well, just about everything. Anything that's made of lots of atoms and molecules all dancing and wiggling around emits thermal radiation. A hot stove emits thermal radiation. Your body emits thermal radiation. A cup of coffee, even a cold one, emits thermal radiation.
Using this blackbody contraption, physicists discovered some important things about the universe. For one, the hotter an object is, the more light it gives off. Second, the hotter it is, the higher-energy light it gives off. A cool cucumber may only emit weak infrared waves, or even weaker microwaves. Extremely hot objects like the surface of the sun will glow in visible light. Even hotter objects spew ultraviolet, or even X-ray, light.
But how?
Even playing field
The problem the physicists were grappling with was one of connection. How does the temperature of an object determine what kind of light it emits? In the prevailing model at the time, a vibrating atom or molecule would divide its energy equally, parsing it out to all the wavelengths of lights possible.
This model is, of course, wrong, because your hot cup of coffee doesn't spit out X-rays into your hand every time you pick it up. But nobody had a better idea.
At the turn of the 20th century, a physicist by the name of Max Planck ... didn't have a better idea, either. Instead, he had a really, really bad idea. He had been kicking around this problem for quite some time, and by his own admission, what he did next was basically an ugly hack, an attempt of last resort to try to find something, anything that could explain blackbody radiation.
His ugly hack was this: He introduced a constant, a single number that connected how energy from inside the walls of the blackbody chamber transformed into radiation.
This number told him how much it "cost" to emit a certain amount of light. You couldn't just spew any amount of any kind of light that you felt like, provided that it eventually added up to the right amount of energy. No; if you wanted to emit some infrared light, it would cost this much energy. And if you wanted to emit something harder, like X-rays, it would cost that much energy. Planck's constant was the link providing the scale between what kind of light you wanted to emit and how much it would cost to make it.
Staying constant
Planck's constant also had another remarkable implication: It said that light could be emitted only in finite, discrete chunks, later called photons. At a given wavelength, there was a smallest amount of light that could possibly exist. You couldn't make half a photon, or 64.4 photons, for example; you could only make whole numbers of photons.
This little hack completely solved the blackbody problem. A warm object has a certain amount of energy available to make radiation. Your coffee might love to make X-rays — but X-rays are expensive, and it can't make half an X-ray. Instead, it has to settle for the budget-friendly infrared radiation.
Planck first proposed this little nugget of an idea in a 1900 paper, and the concept was later picked up by Albert Einstein himself. From there, the idea grew. Perhaps it's not just light itself that comes in discrete, finite packets. Perhaps it's many things. Perhaps reality, at its most fundamental, subatomic level, is … quantized.
That single realization opened the door to what we now call quantum mechanics: that the physics of the very small is based on discrete packets of energy, momentum, and more. It turns out that the rules of the universe at subatomic scales don’t look very much like our macroscopic rules, and we have Max Planck (accidentally) to thank for it.
- Why Can't Quantum Mechanics Explain Gravity?
- Massive Space Structures Have Surprising Connection to Quantum Mechanics
- Black Holes: Everything You Think You Know Is Wrong
Paul M. Sutter is an astrophysicist at The Ohio State University, host of Ask a Spaceman and Space Radio, and author of "Your Place in the Universe" (Prometheus Books, 2018). Sutter contributed this article to Space.com's Expert Voices: Op-Ed & Insights.
Learn more by listening to the episode "Who's in charge of the quantum world?" on the Ask A Spaceman podcast, available on iTunes and on the web at askaspaceman.com. Thanks to Nir Ben Z., Brent R., Frankie C., vicki K., DiaLogical, Katya N., and @fellabear for the questions that led to this piece! Ask your own question on Twitter using #AskASpaceman or by following Paul @PaulMattSutter and facebook.com/PaulMattSutter. Follow us on Twitter @Spacedotcom and on Facebook.
Sign up for the Live Science daily newsletter now
Get the world’s most fascinating discoveries delivered straight to your inbox.
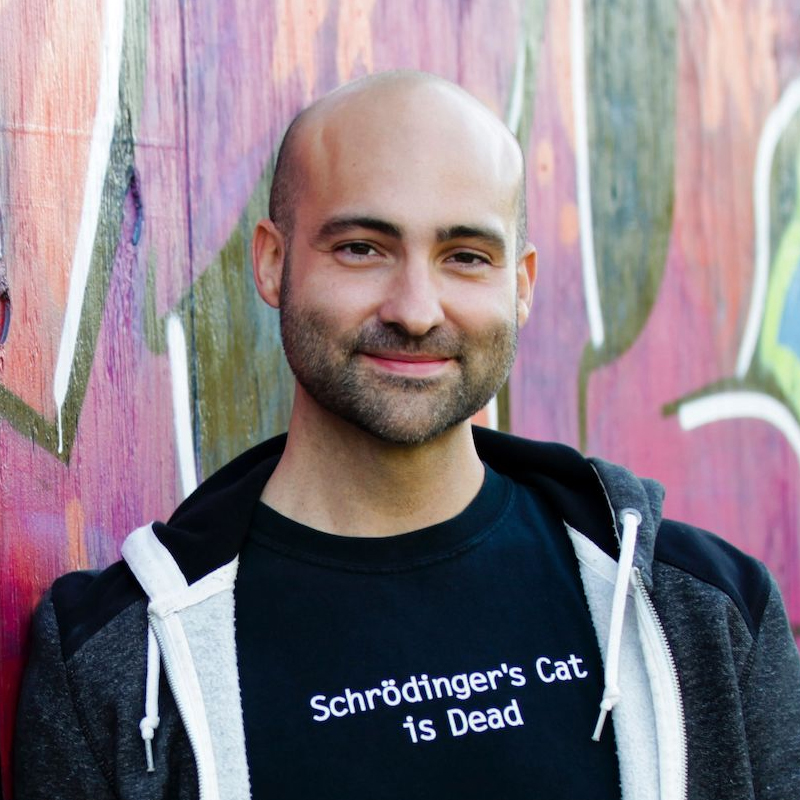
Paul M. Sutter is a research professor in astrophysics at SUNY Stony Brook University and the Flatiron Institute in New York City. He regularly appears on TV and podcasts, including "Ask a Spaceman." He is the author of two books, "Your Place in the Universe" and "How to Die in Space," and is a regular contributor to Space.com, Live Science, and more. Paul received his PhD in Physics from the University of Illinois at Urbana-Champaign in 2011, and spent three years at the Paris Institute of Astrophysics, followed by a research fellowship in Trieste, Italy.