
Tracing the Earth’s Hottest Volcanoes from Core to Ore
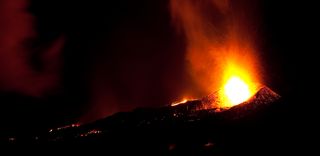
This article was originally published at The Conversation. The publication contributed the article to Live Science's Expert Voices: Op-Ed & Insights.
Volcanic eruptions are as old as the planet itself. They inspire awe, curiosity and fear and demonstrate the dynamic internal activity of the Earth. However, the impact of modern volcanoes pales in comparison to those that graced our planet millions (even billions) of years ago.
These include “supervolcanoes”, volcanic eruptions a thousand times more powerful than the 1980 eruption of Mt St Helens; and large igneous provinces (LIPs), which consist of rapid outpourings of more than one million cubic kilometres of basaltic lava, such as the Siberian Traps in Russia.
In a paper published this week in the Proceedings of the National Academy of Sciences, my colleagues and I set out to find how the hottest and rarest type of volcanoes – the ancient komatiites – were formed.
Knowing how and why komatiites are concentrated in specific belts could help discover new ore deposits, potentially worth billions of dollars.
Komatiite lava flows date back around 1.8 to 3.4 billion years and formed when Earth’s mantle (the layer between the crust and the outer core) was much hotter.
They erupted at temperatures exceeding 1,600C and produced hose-like fire fountains and lava flows that travelled at more than 40km/h as bluish-white, turbulent lava rivers.
Sign up for the Live Science daily newsletter now
Get the world’s most fascinating discoveries delivered straight to your inbox.
These crystallised to form some of the world’s most spectacular igneous rocks – as well as a number of giant nickel deposits, found mainly in Western Australia and Canada.
Komatiites have been studied for more than 60 years and are fundamental in developing our knowledge of the thermal and chemical evolution of the planet, but until recently we didn’t understand why they formed where they did.
So how are komatiites formed?
Komatiites are found in ancient pieces of crust, or cratons, preserved from the Archean Eon (2.5 to 3.8 billion years ago). These cratons contain greenstone belts – preserved belts of volcanic and sedimentary material that often contain deposits of precious metals.
Many cratons exist worldwide. One of the largest is Western Australia’s Yilgarn Craton, which hosts most of the gold and nickel mined in Australia. This craton has only a few specific belts that contain major komatiite flows.
Previous research shows that komatiites were formed from mantle plumes – upwelling pipes of hot material that stretch from the outer core to the base of the crust.
Around 2.7 billion years ago in a huge global event referred to as a “mantle turnover”, multiple mantle plumes formed, and one hit the base of the early Australian continent – the Yilgarn Craton, forming some of the hottest lavas ever erupted on Earth.
When plumes first hit the base of the lithosphere – the 50-250km-thick rigid outer shell of the Earth – they spread out into discs of hot material more than 1,000km in diameter.
Today there is evidence of this in places such as the huge Deccan basalts that cover much of India.
Despite this spread, komatiite belts are sparse and only found in certain areas. One of our research goals was to find out why.
Mapping the early Australian continent
We used specific isotopes of the element hafnium to determine the age of the crust that formed the granites (the material which makes up the cratons) and if it had a mantle or a crustal source.
Mapping out the isotopic compositions of the granites revealed a jigsaw pattern in the crust, and regions where the granites formed by melting pre-existing, much older crustal rocks.
It also showed younger areas where the crust was newly created from sources in the deeper mantle.
By collecting samples of Archean granites from all over the Yilgarn Craton, we were able to map the changing shape of the Archean continent through time.
When we compared the nature and shape of the continent with the location of the major komatiite events, we found a remarkable correlation. The maps showed that the major komatiite belts and their ore deposits were located at the edge of the older continental regions.
This is due to the shape at the base of the ancient Australian continent. As the plume rises, it impacts the older, thick lithosphere first.
As a result the plume cannot generate much magma so it flows upwards along the base of the lithosphere into the shallower, younger areas. Here huge volumes of magma are generated at the boundary between the old, thick and young, thin areas of the lithosphere, so komatiites and their nickel deposits are located at the margins of Earth’s early continents.
Some research questions remain. The origin of the continents imaged in our study and the tectonic system that formed them is still unknown.
What our work shows is that continent growth significantly affects the location, style and type of later volcanism, as well as the location of major ore deposit areas.
We hope that this work will help unravel the volcanic history of other ancient geological terranes, as well as aid in the search for mineral deposits in relatively unexplored cratons such as those in West Africa and central Asia.
This project was funded by Australian Research Council (ARC) Linkage Grants LP0776780 and LP100100647 with BHP Billiton Nickel West, Norilsk Nickel, St Barbara, and the Geological Survey of Western Australia (GSWA). The Lu-Hf analytical data were obtained using instrumentation funded by Department of Education Science and Training (DEST) Systemic Infrastructure grants, ARC Linkage Infrastructure, Equipment and Facilities (LIEF), National Collaborative Research Infrastructure Strategy (NCRIS), industry partners, and Macquarie University. The U-Pb zircon geochronology was performed on the sensitive high resolution ion microprobes at the John de Laeter Centre of Mass Spectrometry (Curtin University).
This article was originally published on The Conversation. Read the original article. Follow all of the Expert Voices issues and debates — and become part of the discussion — on Facebook, Twitter and Google +. The views expressed are those of the author and do not necessarily reflect the views of the publisher. This version of the article was originally published on Live Science.