Can we solve the black hole information paradox with 'photon spheres'?
Are black holes as simple as they appear, or is there more to their story?
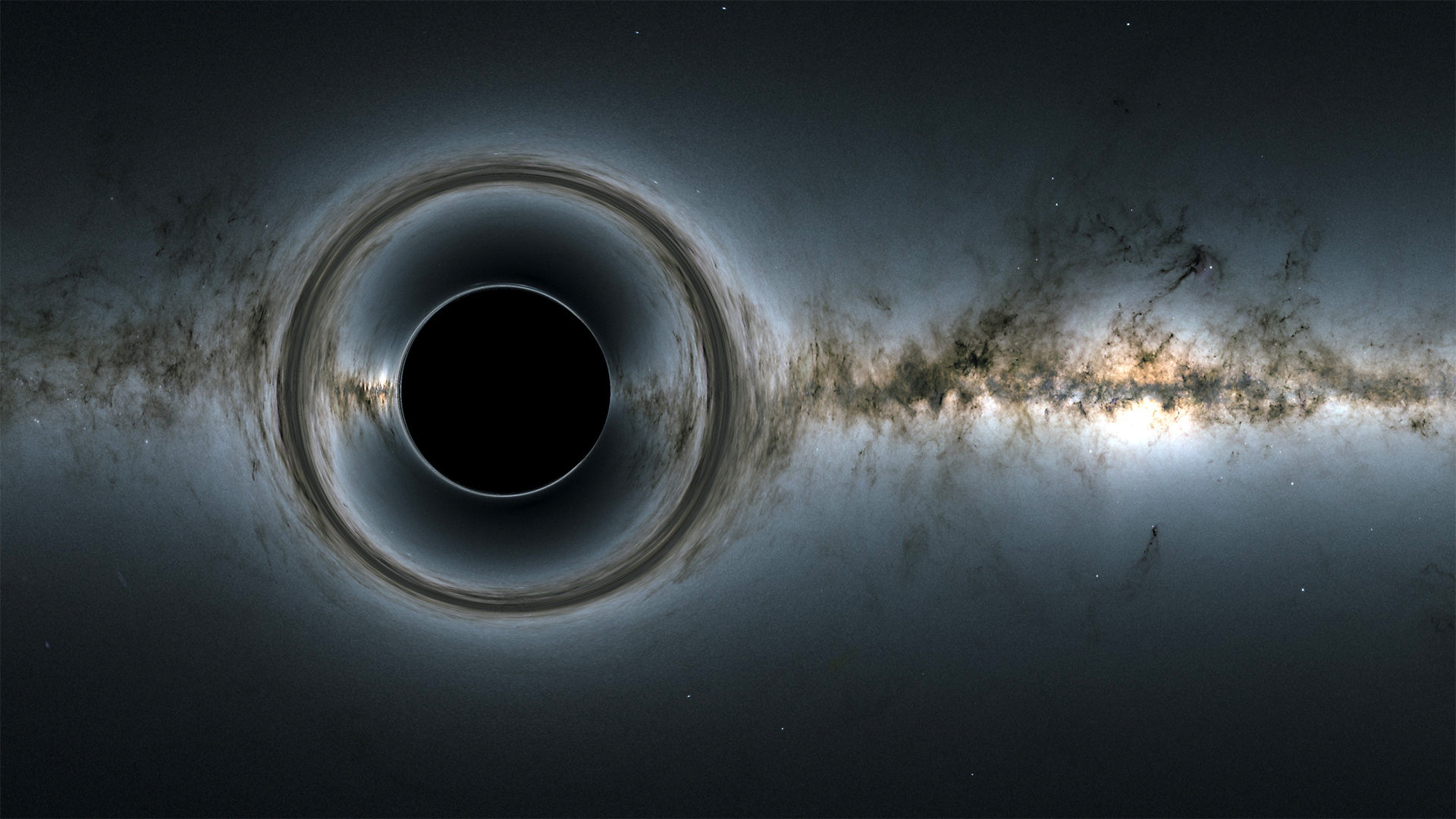
Are black holes as simple as they appear, or is there more to their story?
Theories that attempt to resolve the so-called black hole information paradox predict that black holes are much more complicated than general relativity suggests. Future observations of photon spheres — swirling bands of light around the edges of black holes — will be able to test these theories.
The information paradox
According to Albert Einstein's theory of general relativity, black holes are surprisingly simple. If you know the mass, charge and spin of a black hole, you know everything there is to know about it. In fact, black holes are some of the simplest and most straightforward characters in the universe.
But that apparent simplicity gives rise to a troubling paradox. In the 1970s, famed astrophysicist Stephen Hawking realized that black holes aren't completely black. Instead, they emit radiation through a subtle quantum mechanical process operating at their event horizons, or the boundaries of black holes where nothing, not even light, can escape.
Because black holes are so simple and can be described with only three numbers, all the information about the material that falls into black holes is seemingly locked away forever. It doesn't matter if you build a black hole out of dead stars and interstellar dust or a black hole out of cats; as long as those two black holes have the same spin, mass and charge, they will be identical.
In Hawking's original formulation of his radiation process, that radiation carried no information away with it. But as the black hole emits radiation, it evaporates, eventually disappearing altogether — hence the so-called black hole information paradox. If a bunch of information falls into a black hole, and information can't be destroyed, then when the black hole disappears, where does all the information go?
Video: Scientists find clump of black holes inside the heart of globular cluster
Look to the light
Over the past few decades, there have been many attempts to solve the information paradox. Some of those proposals involve extending our knowledge of general relativity. Some involve attempts to marry general relativity with our understanding of quantum mechanics. And some are just downright strange.
As of yet, however, all attempts to solve the information paradox are unproven. It's very difficult to observe black holes directly, as we usually see them only when they interact with their surroundings (usually by swallowing large clumps of gas or dust) or when they merge and release gravitational waves.
But all that changed in 2019, when a global network of telescopes known as the Event Horizon Telescope worked in concert to deliver a single image of M87*, a supermassive black hole in the center of the Virgo galaxy.
That image is striking and haunting. The dark void at the center is the shadow cast by the black hole's event horizon, preventing any light behind the black hole from piercing through. And that void is surrounded by a ghostly ring of light emitted by the superheated plasma surrounding the black hole.
Ring of fire
What can this image tell us about the true nature of black hole event horizons?
The event horizon of the black hole itself is far smaller than its shadow; the shadow appears so big because of the extreme bending of space near the black hole. And lying somewhere between that event horizon and the edge of the shadow is an interesting feature that also results from the extreme bending of space: the photon sphere. The photon sphere is a region near a black hole where the gravity is so strong that light itself can orbit around the black hole.
The orbits in that region are unstable; the photons can loop around the black hole a few times, but they will not stay forever. Eventually, they will leak out, giving rise to a thin, visible ring of light around the black hole.
In classical general relativity, that photon sphere is so thin that it barely even exists and is far too faint to be seen in the image of M87* taken with the Event Horizon Telescope.
Now, a team of researchers has investigated the properties of the photon sphere in theories that attempt to solve the black hole information paradox. They found that some complex theories of black hole event horizons influence their surrounding environment, including the photon sphere. In some of these theories, it's possible for the photon sphere to be far wider, and thus far brighter, to distant observers, the team wrote in a paper published recently to the preprint database arXiv.
Despite the change, the differences in the photon spheres between classical relativity and the predictions of these exotic models are still too small to be seen with the Event Horizon Telescope. But that telescope's incredible image will not be the last picture we take of a black hole. Future attempts to study M87* and other supermassive black holes will deliver higher-resolution images. If we can resolve the photon ring, studying its width and brightness will give us clues as to the nature of the event horizon and, ultimately, how to resolve the black hole information paradox.
Learn more by listening to the episode "Could we really terraform Mars?" on the Ask A Spaceman podcast, available on iTunes and on the Web at http://www.askaspaceman.com. Ask your own question on Twitter using #AskASpaceman or by following Paul @PaulMattSutter and facebook.com/PaulMattSutter.
Sign up for the Live Science daily newsletter now
Get the world’s most fascinating discoveries delivered straight to your inbox.
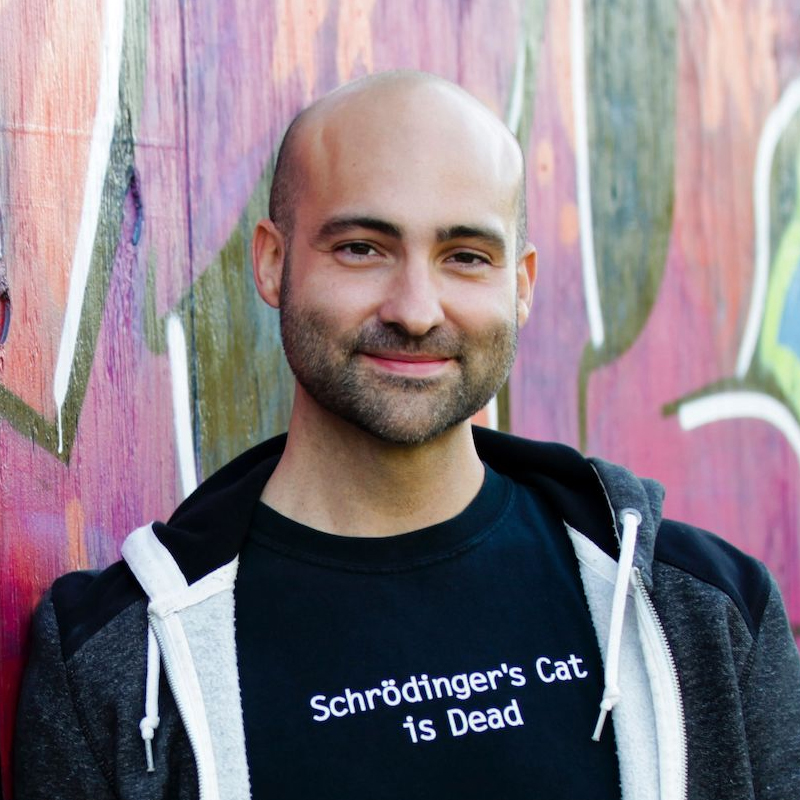
Paul M. Sutter is a research professor in astrophysics at SUNY Stony Brook University and the Flatiron Institute in New York City. He regularly appears on TV and podcasts, including "Ask a Spaceman." He is the author of two books, "Your Place in the Universe" and "How to Die in Space," and is a regular contributor to Space.com, Live Science, and more. Paul received his PhD in Physics from the University of Illinois at Urbana-Champaign in 2011, and spent three years at the Paris Institute of Astrophysics, followed by a research fellowship in Trieste, Italy.